Blue Lasers
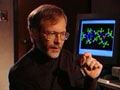
Will Rees
Atlanta (October 1, 2001) — William S. Rees Jr. imagines a future where we will carry all of the information that we would ever need-our driver's license, credit cards, security codes, even our mother-in-law's phone number-on a CD-like disk the size of a dime. With the same data storage capability that would make this possible, we could also put the entire Library of Congress on a single 12-inch disk. The key to this future is the production of a commercially viable blue laser, what Wired Magazine calls "the Holy Grail, the closest the semiconductor biz comes to pure sex."
The Hunt for True Blue
The quest for an efficient blue light-emitting device that could be mass manufactured is not new-a slew of universities and private corporations across the globe have tried for years to manufacture one. Some researchers have achieved limited success, but the same critical problems persist: the lifetimes of such devices are too short; their operating temperatures too high; or, in the case of lasers, they can only operate in a pulsed mode. But according to Rees, who directs the Molecular Design Institute at Georgia Tech and holds a joint professorship in the School of Chemistry and Biochemistry and the School of Materials Science and Engineering, a technique called doping will address all of those challenges.
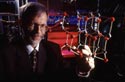
Will Rees
Doping
A chemical process called doping—the addition of impurities to the semiconductor—actually makes the lasing medium a more efficient light emitter. Will Rees' expertise lies in this area of molecular design—the making of the raw materials for a laser that is part science and part art.
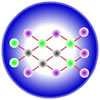
Like the concept for the laser itself, doping has been around for decades. The challenge now for researchers in universities and corporations across the world is to do it best and patent a material-generating process that results in a laser that is efficient and pure enough in color to be used in a widespread setting. Rees calls his particular doping technique "the world's smallest paintbrush" because he designs molecules containing atoms of the dopant which he can place both exactly where, and in what concentration, he desires.
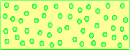
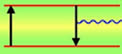
How a Laser Works
The concept behind the laser is simple: when energy (usually in the form of electricity) is pumped into a certain material, it emits light. In 1960, ruby became the first material made to lase. But these days, scientists prefer semiconductors because their unique physical properties make them the best sources of laser light.
Light is composed of photons. If a photon of the right wavelength hits an atom, it will bump one of the atom's electrons up to a higher energy level. (At this point, the atom is said to be in an excited state.) When the electron falls back down, it gives off new light of the same color in a random direction. But if a photon hits an atom that is already excited, that atom emits another photon that is exactly identical to the original: the same color, travelling in the same direction.
When those two identical photons hit two more excited atoms, the result is four, then eight, and so on. The photons of light are amplified. Thus, the word LASER is an acronym for "light amplification by stimulated emission of radiation."
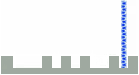
Why Blue?
In a word: wavelength. At around 450 nanometers, blue is shorter than any other color except violet in the visible spectrum. As the graphic on the left illustrates, in the case of data storage, this shorter wavelength allows a blue laser to read and write more information by fitting into smaller "grooves" on an optical disk. A close cousin to the laser, the light-emitting diode (LED), could also have a tremendous impact if made to shine blue. [ A blue LED could be combined with the primary colors of red and green to make any other color, including white, which would open up a whole new world of lighting possibilities. ]
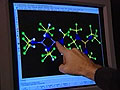
Will Rees
Industry Revolution
In the United States, LEDs are most familiar as lights in car spoilers and personal computer on/off buttons. But a blue LED could be combined with the primary colors of red and green to make any other color, including white. Because white LEDs would be up to 12 times more efficient and longer lasting than conventional light bulbs, experts predict that they will revolutionize the industry, eventually replacing most of the bulbs in the world. The Japanese already employ LED traffic lights, and researchers at Sandia National Laboratories and Hewlett-Packard reported that LEDs could represent a global "cost savings of $100 billion a year . . . and carbon emission reductions of approximately 350 million tons a year (assuming that all the savings come from coal-fired plants)."
A Multi-Billion Dollar Market
The economic potential is certainly not lost on Rees. As a materials chemist, he gets visibly excited by the problem-solving opportunities that his blue laser research presents. The doping that he refers to is simply the controlled addition of "impurities" to the semiconductor, which make it a more efficient light emitter. This materials modification is accomplished through a process called chemical vapor deposition. Rees dubs his particular technique "the world's smallest paintbrush" because he designs molecules containing atoms of the dopant which he can place both exactly where-and in what concentration-he desires. Whereas other methods "paint" only what is in the direct line of sight, Rees works in three dimensions and "gets around the corners, and into all the nooks and crannies."
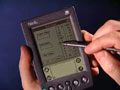
Will Rees
The Molecular Design Institute (MDI) at Georgia Tech is a multidisciplinary research unit within the College of Sciences funded by the Office of Naval Research and the Georgia Research Alliance. Its purpose is to combine the efforts of chemists, biologists, engineers, materials scientists, and physicists in atomic-scale work with new molecules and materials. MDI's mission also includes the education of graduate students, and for Rees, it presents a special opportunity.
"MDI gives graduate students the chance to conduct research which lies at the intersection of science and engineering, and take advantage of the best of what the Georgia Tech research environment has to offer," he says. "Those students are the heart and soul of everything we accomplish. Beyond all of the discussion about future applications of high-tech materials, the students are why I enjoy what I do."
For more information contact:
Megan McRainey, Communications & Marketing
Contact Megan McRaineymegan.mcrainey@comm.gatech.edu
404-894-6016